Energy Harvesting's Emerging Role in a 'Smarter' World
- Christian Pennisi
- Sep 1, 2016
- 6 min read
(Original Published in NASA Tech Briefs)
When most individuals hear “energy harvesting,” they often think of alternative energy sources like wind and solar power. There is a distinct difference, however, between alternative energy and energy harvesting, or EH, approaches, based on the amount of power each can generate.
Alternative energy commonly focuses on grid off-set methodologies aimed at reducing fossil fuel usage. For example, wind turbines are capable of producing up to three megawatts per hour, enough to power almost 500 homes. Energy harvesting, on the other hand, typically produces energy in the microwatt range and is not considered a viable candidate for grid use.
So, why even bother with energy harvesting? Although there are many obstacles to its mainstream use, energy harvesting has an opportunity to support a growing number of devices and objects as they become connected to the Internet.
As an example, let’s consider a kitchen wall clock. Sure, two AA batteries will keep a traditional clock running for a few years. Now, think about the benefits of a clock that provides digital weather alerts in real-time and connects with mobile devices via Wi-Fi to offer texts, calls, and other push notifications. Suddenly, those two AAs cannot be expected to power the clock for extended periods of time, given the constant battery drain posed by the new “smart” clock features.
Enter Energy Harvesting
Instead of powering clocks with batteries that need continual changing, that new clock can now run non-stop for decades by combining a thermal gradient harvester with small solar cells. A thermoelectric energy harvester uses two dissimilar materials to convert a temperature difference into energy.
The same general concept surrounds the Internet of Things (IoT), which connects vast numbers of inanimate objects extrapolating copious amounts of realtime data to create increased operational efficiencies, in-depth insights, and human-free automation.
Imagine vital signs monitored with the accuracy needed to notify medical staff days in advance of a pending heart attack, or fully automated manufacturing plants operating with machines that repair themselves with no unplanned downtime.
IoT ideas perhaps sound complicated. In reality, however, these scenarios actually include a very simple process chain: data is collected by sensors; that data is transferred to either a fog or cloud network; the information is processed; and either extrapolated information or actuation commands are dispersed.
Where does energy harvesting fit into the mix? Go back to the clock example. In order to provide digitally-aware insights 24/7, the clock needs three components: the right software, the right hardware, and a steady, consistent power source. The same is true for widespread IoT implementations. Some updates would only require the latest software, with other applications needing new hardware integrated into the operational environment.
Opting for energy harvesting as a power source drastically reduces the initial and ongoing maintenance and cost of modular IoT upgrades, as well as offers a quicker and more simplified installation method. It may not seem too troublesome to change out the batteries of one clock, but imagine the effort involved in replacing and maintaining the batteries on tens of thousands of sensors and nodes installed throughout a manufacturing plant. The process would take months, if not longer, to complete the seemingly innocuous task.
In addition to time-saving benefits, energy harvesting also provides increased data rates for IoT applications. Real-time data is often the goal of many operations, and in some scenarios an absolute necessity. The energy cost for real-time, non-stop data collection and transmission is extremely high. Battery-powered sensors or transmitters operating in real-time, as opposed to delayed iterations, require more frequent battery replacements. As a result, efficient energy harvesting power sources would be needed at the data collection point, with wireless transmission and increased security features integrated into the sensor itself.
Finally, another energy harvesting benefit is mobility. A clock can be simply plugged into an outlet, provided an outlet is nearby. When the clock is self-powered, however, it can be placed virtually anywhere. This is a very important advantage in the IoT realm, where sensors or nodes are many times placed in remote, mobile, or hazardous locations, making hard-wiring or the regular replacement of batteries extremely cumbersome.
The Obstacles
Why hasn’t implementation happened already? There are successful energy harvesting IoT technologies that exist currently, but they are largely focused on very specific applications. In order for energy harvesting to reach its widespread implementation potential, there are a few barriers that still have to be breached.
Power Generation
Energy harvesting is generally talked about in terms of power creation relative to size, with the standard being cm2. Most energy harvesting products within the landscape typically generate energy in microwatt range (μW). The amount of power can be valuable for MEMS sensors and a few other potential niches, but not for realizing the full potential of energy harvesting in the IoT marketplace.
The current ideal power range for operating sensors and nodes is around 10 milliwatts (mW). Unfortunately, such a capacity is still a stretch for most energy harvesting platforms. The most prevalent energy harvesting platforms include thermoelectric, piezoelectric, RF harvesting, photovoltaic, and electrodynamic devices.
Thermoelectric harvesters convert a temperature difference into energy, but the devices only reach significant outputs when coupled together.
Piezoelectric crystals, used as vibrational energy harvesters, generate energy when their physical state is altered. In order to maximize the amount of created energy, however, the harvester must be tuned to the vibration of the desired installation site, which becomes a drawback for the goal of widespread implementation.
RF energy harvesting converts ambient radio frequency waves into energy. While wireless energy is the most ideal scenario, RF harvesters tend to generate the lowest amount of usable energy.
Currently photovoltaic and electrodynamic technologies have the highest power generation. Photovoltaic devices are capable of harvesting 100 mW/cm2 with outdoor light, but only 100 μW/cm2 with indoor light. Additionally, most energy harvesting technologies need to act as embedded components within enclosures that do not easily enable the collection of light for power.
Electrodynamic energy harvesting uses the basic principle of magnetic induction to harvest energy from motion and generate power in the mW range. While there are models that harvest from a wide variety of motion sources, however, many of the variations are bulky, again drawing concerns as embedded solutions.
Intermittency
Intermittency is also a challenge for users implementing energy harvesting platforms. Just like with alternative energy, the consistency of freely harvested energy can be difficult to guarantee. Think of solar panels. As long as the sun is shining brightly, there is plenty of available energy.
Similarly, every energy harvesting method faces the same threat of intermittency. There is no way to guarantee the consistency of power output, since consistency from the harvesting source is also not guaranteed.
ROI
Finally, and possibly the biggest hurdle yet for mainstream energy harvesting use, is the ability to present data-backed ROI projections. Energy harvesting benefits must be clearly demonstrated. Of course, there are and will be unique scenarios in which EH proves to be the best solution. To beat out alternate power sources, it will take real data from pioneering companies who want to implement energy harvesting into the early stage development of their overall platforms.
A Pathway for Energy Harvesting
Collectively, the hurdles point in the direction of one best-case option overall: a small, low-cost, battery-coupled, vibration- based, electrodynamic energy harvesting platform. The smaller the device, the more easily it can be integrated. In addition, low cost is absolutely necessary for widespread implementation.
With this form of energy harvesting, the issue of intermittency is overcome by coupling a battery and energy harvester together. The battery acts as a primary power source, while the energy harvester keeps the battery charged to provide an elongated lifespan. With current battery developments, 20+ years of operation is not unreasonable.
Finally, vibration and electrodynamics go hand-in-hand. Whereas piezoelectric vibrational harvesting requires a “tuning” element, it is theoretically possible that an electrodynamic vibration model could operate without the need for major individual adjustments.
Essentially, the only current factor preventing the technology from reaching further into the IoT space is scale. For the many energy harvesting devices that meet the necessary size requirements, power density is often a challenge. So, either we wait for a smaller vibrational source with a high enough energy density, or a combined energy-harvesting, battery-powered technology that collectively meets both power needs and necessary lifespan.
As a result, an electrodynamic, vibrational energy harvester with battery power management could prove an extremely important pathway to the widespread implementation of Internet-of-Things technologies, regardless of industry. In such a world, software updates on open platform systems will be the norm (with modular hardware upgrades). Energy harvesting could provide cost-effective methods for scaling up the physical side of IoT systems worldwide.
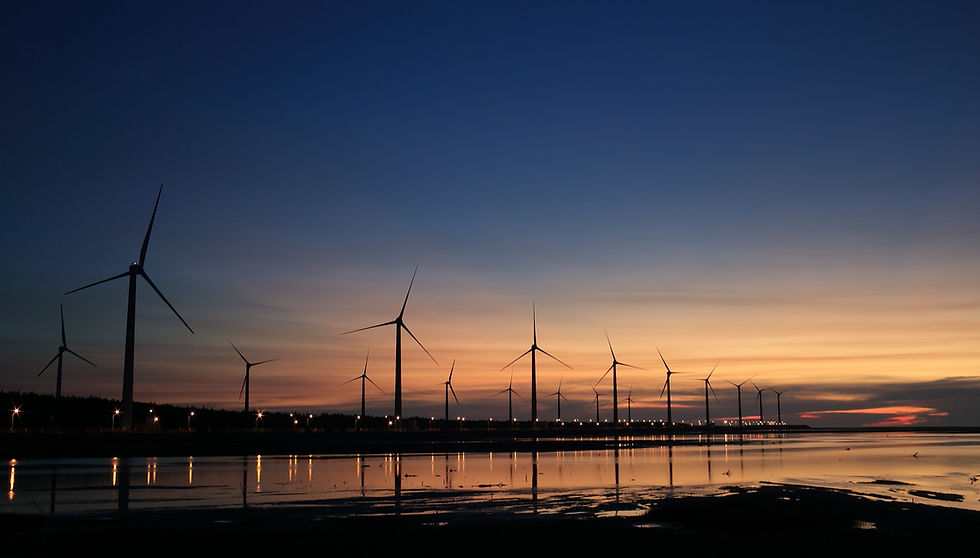
Comments